​
Lipid-induced insulin resistance
For the past fifteen years we have focussed heavily on assessing the impact of cellular over-nutrition (as is commonly observed in obese individuals who maintain a state of positive energy balance) upon insulin sensitivity in tissues such as skeletal muscle. In particular, our work has focused on establishing how over-provision of free fatty acids (e.g. palmitate) induce defects in insulin signalling and consequently lead to impaired physiological control of key cellular processes regulated by the hormone, such as, for example, the uptake and metabolism of glucose. However, as yet, there is no unified view that adequately explains how these pathophysiological events develop.
Randle postulated over 50 years ago that increased mitochondrial fatty acid oxidation (associated with fatty acid oversupply) may impair glucose uptake and utilisation via metabolite-induced allosteric inhibition of key glycolytic enzymes. However, the concept that suppressed glycolytic flux underlies lipid-induced metabolic dysfunction has been challenged by an alternative school of thought that suggests ectopic accumulation of lipids as the primary cause of insulin resistance in skeletal muscle. In particular, oversupply of excess saturated fatty acids (e.g. palmitate) has been linked to intramyocellular accumulation of bioactive lipids such as diacylglycerol (DAG) and ceramide. These lipids have been implicated strongly in the development of insulin resistance via their inhibitory effects on proximal insulin signalling. Notably, activated DAG-sensitive PKCs suppress insulin action via elevated serine phosphorylation of insulin receptor substrates, such as IRS-1, whereas ceramide induces a targeted loss in activation of the serine/threonine kinase, Akt by insulin. Both IRS and Akt serve as critical nodes in the insulin signalling network and so their dysregulation impairs key processes under the control of insulin, including glucose uptake and glycogen synthesis.
In addition to the Randle hypothesis and the notion that lipotoxic intermediates impact negatively on insulin signalling, there is also growing appreciation of the relationship between insulin sensitivity and mitochondrial function. Importantly, various indicators of impaired mitochondrial capacity have been reported in skeletal muscle from insulin-resistant and/or obese human subjects including, for example, a reduction in the activity and/or expression of carnitine palmitoyltransferase-1 (CPT-1), which mediates mitochondrial fatty acid uptake, and that of various oxidative enzymes and respiratory chain components coinciding with suppressed ATP synthesis. Recent work from the Hundal lab has assessed the importance of mitochondrial function as a determinant of insulin sensitivity and the contribution that it may also make towards fatty acid-induced proinflammatory signalling. Specifically, we have tested the idea that mitochondrial dysfunction associated with substrate/fuel overload is a crucial determinant of insulin resistance/tissue inflammation.
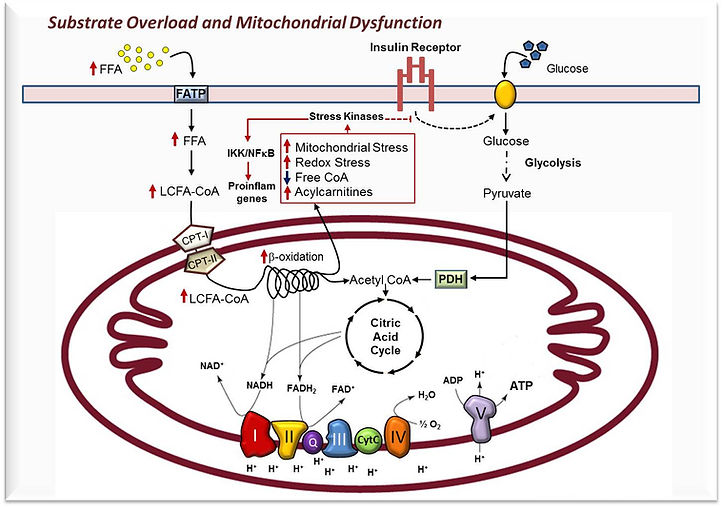
Our studies have revealed that muscle cells incubated with palmitate (or indeed a mixture of fatty acids at concentrations that may circulate in plasma during obesity) and physiological concentrations of glucose lead to a (i) reduction in Akt-directed insulin signalling, (ii) reduction in insulin-stimulated gene expression and (iii) enhanced activation of NFkB signalling and proinflammatory cytokine (IL6, CINC1) gene expression. These events are associated with a reduction in mitochondrial fatty acid oxidative capacity, a decline in cellular respiration (assessed by measuring cellular oxygen consumption) and a fall in the expression of OXPHOS proteins (cytochrome C oxidase, succinate dehydrogenase (SDHA)) and that of PGC1-alpha (a regulator of mitochondrial biogenesis). These latter responses are all highly suggestive of a reduction in mitochondrial integrity and function and, indeed, consistent with the reduction in SDHA, mass-spec metabolite analysis reveals a dramatic decline in fumarate (the product of succinate oxidation by SDHA) and malate, which is generated from hydration of fumarate. Strikingly, these changes in mitochondrial integrity/function are not seen in muscle cells incubated with palmitate alone (i.e. no glucose) or in which glucose metabolism is inhibited using a glycolytic inhibitor (2-deoxyglucose). Importantly, under these circumstances we find palmitate is no longer able to promote a reduction in Akt-directed insulin signalling and palmitate’s proinflammatory drive is also substantially muted. The physiological “health” of the cells under these conditions is critically dependent upon being able to utilise palmitate as an oxidative fuel. However, the ability to oxidise palmitate in the absence of glucose requires sustained generation of key TCA metabolites such as oxaloacetate (OAA), via anaplerotic reactions such as that catalysed by pyruvate carboxylase. As anticipated, cells incubated with palmitate alone exhibit a dramatic reduction in glycolytic intermediates, such as G6P and glyceraldehyde 3-phosphate). Intriguingly, however, we find that despite withdrawing glucose the abundance of pyruvate (the end-product of glycolysis) is maintained in myotubes treated with the fatty acid alone. We postulated that in the absence of glucose, pyruvate is generated from alanine (via transamination) and that the irreversible carboxylation of pyruvate by pyruvate carboxylase would then support mitochondrial anaplerosis. In line with this hypothesis, inhibitors of alanine aminotransferase or pyruvate carboxylase reduce palmitate utilisation and, despite the absence of glucose, insulin resistance and proinflammatory signalling are reinstated.
These findings are not simply a facet of the cultured muscle cell line that we use. We have found that suppressing glucose utilisation (by using 2-deoxyglucose as a dietary supplement) in obese insulin resistant rodents induces a marked improvement in muscle insulin sensitivity (based on enhanced Akt activation) and a reduction in muscle proinflammatory tone – based on reduced muscle IL-6 and CINC-1 mRNA expression. Collectively, our observations indicate that mitochondrial function is a crucial regulator of insulin sensitivity and the NFkB pathway and raises the possibility that restraining glycolytic flux could help restrain the excessive carbon load placed upon mitochondria during obesity and by doing so help promote beneficial metabolic changes (i.e. reduced inflammatory signalling and improved insulin sensitivity). It is important to point out that other groups have shown that inhibiting fatty acid entry (and hence influx of fatty acid-derived carbon) into mitochondria when glucose is available also averts the insulin-desensitising effect associated with fatty acid over-provision. Together, these observations imply that, irrespective of which metabolic fuel is available, as long as the total or combined fuel load does not exceed mitochondrial oxidative capacity and respiratory drive is maintained within a normal threshold, the fidelity with which insulin activates molecules such as Akt is likely to be upheld.
As indicated above, saturated fatty acids also induce a proinflammatory response in skeletal muscle, which, in addition to generation of lipids such as ceramide, may also be a contributing factor in the pathogenesis of insulin resistance. How this inflammatory response is initiated is poorly understood, but our studies suggest that saturated fatty acids induce activation of MEK/Erk signalling and that this appears crucial in supporting the downstream activation of the NFkB pathway and the concomitant increase in the expression of proinflammatory genes. Intriguingly, we have discovered that this inflammatory response is antagonised by unsaturated fatty acids. Precisely how this protective anti-inflammatory effect is conferred is unknown, but our on-going work is currently directed at delineating the mechanisms involved.
For more information on our work in this area click on the links to following Journals.